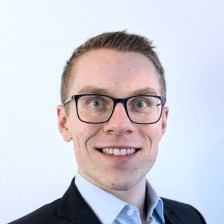
Research
This is an overview of research interests and experimental methods. Visit my blog to see individual publications.
Conjugated polymers
Polymer materials, commonly called “plastics”, can be found in cheap everyday products (toothbrushes, bottles) as well as in advanced applications (airplane parts, eyeglasses). The popularity of polymers is based on their simple processability, tunable mechanical properties, and low cost. Polymers are large, chain-like molecules, potentially consisting of thousands of linked units. If their backbone contains alternating carbon single- and double-bonds, the resulting delocalized π-electron system enables interaction with visible light [1]. This material class, called conjugated polymers, is under active research to enable solution-processed semi-transparent solar cells and flexible displays.
Fig. 1: Chemical structure of the conjugated polymer MEH-PPV. The conjugated backbone is highlighted in blue.
Structure-Function-Relationship
When deposited onto a substrate, conjugated polymers tend to crystallize via attractive van-der-Waals forces [2]. The closeness of the chains then causes electronic interaction and changes the optical properties, such as the absorption and emission spectrum, and the excited state lifetime. The effects are typically interpreted in the H- and J-aggregate model [3], which explains why isolated molecules, solutions and films all show different properties [4]. This structure function relationship can be exploited to optimize device performance via manipulation of the nanoscale morphology [5].
Fig. 2: Conjugated polymers tend to form semicrystalline films (right) instead of amorphous films (left). Crystalline regions are highlighted in blue.
Exciton transport
Sustainable energy generation is arguably the most pressing topic of our lifetime. Organic solar cells (OSCs) may extend the range of available solutions with cheap, flexible, transparent, and integrated solar panels [6]. However, current OSCs are not yet competitive in terms of price, longetivity and efficiency. Unlike conventional silicon based solar cells, OSCs need two materials (called donor and acceptor) to create current. Photon absorption in the donor material creates an excited state, called exciton, that only separates into electron and hole after successful diffusion to the donor-acceptor interface [5]. The low exciton diffusion length (typically 10nm) is therefore a hurdle for efficient device manufacturing. Improving exciton diffusion, e.g. by enhancing the materials crystallinity, may thus enable higher OPV efficiencies and ultimately lead to commercial success.
Fig. 3: Charge separation in a bilayer organic solar cell. The exciton diffusion process is highlighted in blue.
Optical Spectroscopy: Experimental techniques
In my research, I employ spatially, spectrally, and time-resolved optical spectroscopy. In a home-built confocal microscopy setup, we investigate discrete spots on the sample (spot size 500nm) with a focused laser beam. We select “where to look” with a piezo stage, which is also used to scan areas of up to 80x80µm. The spots’s fluorescence is collected with an objective and analyzed with various detectors. For example, we spectrally disperse the fluorescence to obtain fluorescence spectra, with sensitivity sufficient for the analysis of isolated single molecules. Alternatively, we use a TCSPC system to determine fluorescence lifetimes. More experimental techniques are unlocked by scanning the excitation wavelength, using an additional heating stage, a white light lamp, or by using a Bertrand lens to investigate the Fourier space. The vast flexibility in our lab allows us to combine techniques at will to tackle the research question at hand.
Fig. 4: Basic scheme of our home-built confocal microscopy setup.
Collaborators & People
Some people I enjoy working and discussing with:
- Prof. Richard Hildner, Ioannis Touloupas, Asena Cerhan Haink, Eric Woering, Xinpeng Xu, and Foppe de Haan from the OSFN team in Groningen
- Felix Wenzel, Dr. Hannes Welz, Dr. Klaus Kreger, and and Prof. Hans-Werner Schmidt from the chair Macromolecular Chemistry I in Bayreuth
- Dr. Bernd Wittmann, Sebastian Baderschneider, Dr. Kevin Wilma, Dr. Dominic Raithel, and Prof. Jürgen Köhler, my former colleagues at the chair for Spectroscopy of Soft Matter in Bayreuth
- Dr. Björn Kriete, Nong Hoang, Benedito Raul, Sundar Raj Krishnaswamy, and Prof. Maxim Pshenichnikov from our sister group OCMP in Groningen
Literature
-
Heeger, A. J. Semiconducting Polymers: The Third Generation. Chemical Society Reviews 2010, 39 (7), 2354. https://doi.org/10.1039/b914956m. ↩︎
-
Noriega, R.; Rivnay, J.; Vandewal, K.; Koch, F. P. V.; Stingelin, N.; Smith, P.; Toney, M. F.; Salleo, A. A General Relationship between Disorder, Aggregation and Charge Transport in Conjugated Polymers. Nature Materials 2013, 12 (11), 1038–1044. https://doi.org/10.1038/nmat3722. ↩︎
-
Spano, F. C.; Silva, C. H- and J-Aggregate Behavior in Polymeric Semiconductors. Annual Review of Physical Chemistry 2014, 65 (1), 477–500. https://doi.org/10.1146/annurev-physchem-040513-103639. ↩︎
-
Raithel, D. Disorder Phenomena in Conjugated Oligomers and Polymers. PhD Thesis, University of Bayreuth, 2018, https://epub.uni-bayreuth.de/3669/ ↩︎
-
Brabec, C. J.; Heeney, M.; McCulloch, I.; Nelson, J. Influence of Blend Microstructure on Bulk Heterojunction Organic Photovoltaic Performance. Chem. Soc. Rev. 2011, 40 (3), 1185–1199. https://doi.org/10.1039/C0CS00045K. ↩︎
-
Li, N.; Brabec, C. J. Washing Away Barriers. Nature Energy 2017, 2 (10), 772–773. https://doi.org/10.1038/s41560-017-0011-1. ↩︎